Physiology of pleural space after pulmonary resection
Physio-pathology of pleural space
The pleura is a serous membrane which includes the lungs and the adjoining structures. It is formed by two membranes: the visceral and the parietal ones. The two membranes continue one on the top of the other at the hilum and are separated by a thin film of serous liquid (≈0.3 mL/kg) (1,2).
The visceral pleura is the inner one and covers the lungs, blood vessels, bronchi and nerves. It is vascularized by the arterial, venous and lymphatic vessels of the lungs and bronchi. It receives its innervation from the autonomic nervous system and has no sensory innervation. In fact, only the parietal pleura has pain sensitivity. The parietal pleura almost completely covers the costal surface of the thoracic wall, of the mediastinum, of the diaphragm and it forms the pleural domes at the level of the upper thoracic strait. It is divided into three parts: costal, diaphragmatic and mediastinal. It is vascularized by the arterial, venous and lymphatic vessels of the chest wall and innervated by the phrenic and intercostal nerves (3). The pleural sinuses are pleural cavity recesses created by the pleurae folding and they are: the costo-phrenic, the costo-mediastinal, the azygoesophageal and the aortic-esophageal recesses. They contribute to the movement of the lungs during the breathing.
The left and right pleurae are not connected. In fact, in the case of pleural effusion it could remain localized in a single pleural cavity.
The pleura consists of a single layer of mesothelial cells resting on a sheet of loose connective tissue containing collagen, elastic fibers, blood and lymphatic vessels, nerves and cells with the characteristics of fibroblasts (4,5). Epithelial cells present numerous microvilli on their surface that favor the reabsorption by pinocytosis of proteins and the sliding of the two pleural sheets. The phospholipids that are released by exocytosis from the mesothelial cells, are similar to those present in the alveolar surfactant and perform a lubricating action that facilitates the movements of pleural sheets (6).
This mechanism has a fundamental role in preventing injury during the pleurae sliding. The mesothelial cells have also an important role in the defence of the lung. In fact, they have multiple pattern recognition receptors, activated by injuries or bacterial presence, and they are able to produce cytokines and chemokines, such as IL-8, IFNs, TNF and MCP-1. These molecules recruit neutrophils and mononuclear cells, that enter through gap junctions between the cells of the pleural sheets. Those cells also express adhesion molecules, such as ICAM-1, in order to keep the white blood cells in the pleural space. The pleural fluid participates at the defence mechanism too. It contains immunoglobulins (i.e., IgA and IgG) and complement. The pleurae also play a role in the resolution of inflammation (7-12).
The pleural cavity is a virtual space, containing lubricating fluid that allows the visceral and parietal sheets to slide one over the other. In order to fully understand the pleural alteration caused by a pulmonary resection it is important to explain the mechanism by which the pleural space is kept free from air and liquid. The turnover of the pleural fluid is ensured by the filtration of the capillaries and by the lymphatic drainage at the level of the parietal pleura.
The pleural fluid is a plasma filtrate produced by the flowing of the parietal pleura circulation. The visceral pleura does not participate to this process because of its thickness (visceral permeability is 10-fold lower than the parietal one). The pleural fluid contains a low protein concentration (<1.5 g/dL), mainly albumin, glucose and electrolytes. While in the serum there is a higher content of Na and Cl, in the pleural fluid the concentration of HCO3 prevails. The glucose content is similar in the two fluids. A small number of cells (mainly white blood and mesothelial cells) can be found (1,700 cells/mm3) (13-16).
Among its roles, pleura helps the mechanical coupling between the lungs and the chest wall. In order to make it work efficiently the liquid volume should be negligible. During the inspiration, in fact, the visceral pleura follows the thoracic wall movements and this is allowed by the presence of a sub-atmospheric pressure within the pleural cavity. From the base to the top of the pleural cavity the pressure of the pleural liquid (Pliq) becomes more sub-atmospheric, being about zero at the bottom and ∼−10 cmH2O at mid-heart level. The pressure is made more sub-atmospheric by the drainage of the lymphatics. Since the pressure of the pleural fluid is more sub-atmospheric than the elastic return force of the lung, corresponding to the “pleural surface pressure” (Ppl), the visceral and the parietal pleurae push one against the other. At the functional residual capacity, Ppl is ∼−4 cmH2O at mid-heart level. Ppl depends on the elastic capacity of the lung: in fact approaches to zero in an emphysematous lung and becomes more negative than −4 cmH2O in a fibrotic lung (17-20).
The pleural fluid is reabsorbed by the lymphatic system. The liquid filters through pores of 0.3–40 µm of diameter and it runs into the lymphatic vessels, organized into two anastomosed webs (21,22). The vertical distribution of Pliq pressure does not fully reflect a hydrostatic situation. It varies with height because of gravity and intrapleural fluid circulation. Thus, there is a little flow from the top to the bottom, which minimally affects the hydrostatic gradient. At the top of the cavity the sub-atmospheric pressure causes microvascular filtration from the capillaries of the parietal pleura into the pleural space (23,24). This pressure is stronger than the one exerted by the elastic forces of the lung and ribcage for the spring-back, and it keeps the visceral and parietal pleura in close apposition. In this scenario it is important to underline that actually the most important flow is the one which follows a pathway from the costal to the mediastinal and diaphragmatic regions, richer in lymphatics. The reabsorption velocity is in the order of 2 mm/min but it can be markedly increased (in the order of 40 times) to prevent fluid accumulation. Pleural fluid turnover is estimated to be 0.15 mL/kg/h and the fluid renewals in about 1 h (15).
Pleural effusion
When the pleural fluid production exceeds its reabsorption, it tends to accumulate in the pleural cavity. This accumulation is called pleural effusion (15). The pleural effusion is usually classified as exudative or transudative, based on the chemical characteristics. A content of proteins superior to 3 g/dL and a density greater than 1.016–1.018 are considered exudative. In some alterations, the effusion could have mixed features. The pleural effusion is usually a negative prognostic factor which increases the morbidity and mortality of the underlying disease.
According to the Starling law (Figure 1), there are several mechanisms by which the pleural fluid volume can expand between the pleurae:
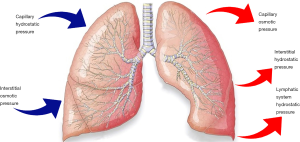
- Increased hydrostatic pressure: both arterial and venous hypertension can exceed the capillary capacity and cause excessive flow through the pleurae. The main causes of increased hydrostatic pressure are congestive heart failure, superior vena cava syndrome, portal hypertension and pulmonary hypertension. This type of effusion is mainly a transudative;
- Reduced intravascular oncotic pressure: albumin is the main protein responsible for keeping the oncotic plasmatic pressure. This protein is produced by the liver, so it can be decreased because of hepatic diseases or a severe state of malnutrition. Moreover, it can be lost with urine in the context of a nephrotic syndrome, through an altered intestinal permeability in some enteric pathologies, through the injured burned skin, or in a condition of myxoedema. Hypoalbuminemia is also a consequence of an increased metabolism, in a malignancy syndrome. This is typically a transudative effusion;
- Increased intrapleural oncotic pressure: this situation is caused by a persistent pleural effusion that can attract further accumulation of transudative fluid;
- Reduced intrapleural negative pressure: this condition is known as “trapped lung”. Because of a diffuse condition of atelectasis (due to a respiratory flow obstruction or a contraction from fibrosis) the lung cannot completely expand, so the negative pressure of the pleural cavity decreases. The effusion is usually a transudative;
- Reduced lymphatic drainage: the lymphatic vessels can be constricted by malignancy, lymphadenopathy, carcinomatosis or yellow nails syndrome. The flow can also be discontinued because of a trauma. This is mainly an exudative or a mixed effusion;
- Increased capillary permeability: this can be due to an injury (a direct or indirect trauma) or, more frequently, to a context of lung or pleural inflammations, infections, mesenchymal diseases, pulmonary infarction or embolism, sarcoidosis, malignancy, drugs reactions, hypersensibility or uraemia. This kind of effusion is usually an exudative or it can have mixed characteristics;
- Altered permeability of the pleural membranes: in a context of pulmonary oedema (mainly consequence of a heart failure), the fluid can flow across the visceral pleura into the pleural cavity. It is typically a transudative;
- Microperforated extravasation across the diaphragm: microstructural diaphragmatic or lymphatic defects permits the flow from a peritoneal effusion during hepatic hydrothorax, cirrhosis, intra-abdominal abscess, peritoneal dialysis, pancreatitis and cholecystitis. This kind of effusion is usually an exudative.
Although it can be caused by all of these mechanisms, the most frequent are: congestive heart failure, pneumonia, malignancy and pulmonary embolism. (13,24-29).
Pneumothorax (PNX)
PNX is characterized by the presence of air or gas in the pleural space. When the parietal or visceral pleura is breached the pleural cavity is exposed to a positive atmospheric pressure, the air will enter this space and the lung will collapse. Any condition that impairs the structural integrity of the pleural membranes can produce a PNX:
It can be classified in three categories:
- Spontaneous: it occurs in patients without a history of any event known to cause PNX. It is observed in young people and it seems to be related to rupture of small peripheral apical sub-pleurical blebs;
- Iatrogenic: it may happen after invasive thoracic procedures such as thoracentesis, transbronchial lung biopsy and subclavian vein catheterization. Although, it may complicate any invasive procedures involving neck or abdomen;
- Traumatic: it is caused by a penetrating or no penetrating chest trauma. While the first one may be easily diagnosed, the second one should prompt a careful search for ribs fracture, oesophageal injuries and bronchial rupture (30-32).
Modifications after pulmonary resection
In the treatment of several lung diseases, a radical or partial lung resection may occur. Though thoracic surgery can solve or improve the symptoms, it alters the physiological mechanical and fluid setting and the water balance of the lung and the pleurae (33). The main conditions that may need a thoracic surgery are chronic obstructive pulmonary disease (COPD), emphysema, air blebs, lung cancer, PNX and mesothelioma.
There are several surgical procedures and approaches used to treat these conditions. The most common types are:
- Pneumonectomy (removal of an entire lung);
- Lobectomy (removal of an entire lobe);
- Sublobar resection (removal of part of a lobe);
- Segmentectomy (removal of a segment);
- Wedge resection (removal of a triangle-shaped slice of tissue);
- Pleurectomy (removal of a part of pleura).
During the post-surgery course, it is necessary to drain the pleural cavity. However, the complete removal of the gas is one of the main causes of the residual lung over-distention, which can lead to severe consequences. Thus, it is necessary to drain the pleural fluid with an adequate suction pressure.
Two different drainage phases can be identified:
- Right after closing the thorax: this allows the air removal and promote lung re-expansion. In this phase there is an increased risk of hydrothorax, air leak and lung oedema;
- A postponed stage when the pleural fluid has accumulated substituting the air bubble: in this phase there is an increased risk of hydrothorax, that should be avoided.
The initial drainage of the gas is performed by opening the thoracic tube positioned in the retrosternal region where the air collects during the supine posture. Vice versa, in the presence of hydrothorax, the pleural fluid is drained in an effective way with the tube positioned in the lower part of the pleural space (costo-diaphragmatic dorsal sinus) (33-35).
A single drain is used by most of surgeons to remove both the air and the pleural fluid (34).
In 2011, with the aim to reach a uniform definition of classification and management of pleural space after lung surgery, the most important European and American Thoracic Surgery Societies published a joint position paper (20).
The terms “active drainage” and “passive drainage” have always been subjects of discussion and misunderstanding. Briefly, it should be described an “active drainage” when a sub-negative pressure from the outside is applied to the pleural space through the use of a pump (siphon effect), and a “passive drainage” when inner pressure is higher than atmospheric one. To simplify the distinction between the two terms, “external aspiration applied” may be used in the case of an “active drainage”, and “no external aspiration applied” in the case of a “passive drainage” (36).
According to these statements two main kinds of suction may be considered:
- Regulated (variable) suction: an external active suction capable of adapting its negative pressure depending on the variability of internal pleural pressure. This drainage manages to keep the pressure value inside a well-defined scale of values;
- Unregulated (fixed) suction: this drainage is still connected to an external suction, but it does not modify its pressure according to the intrapleural changes (37).
Anatomic modifications
In the post-surgical period, lungs, pleurae and pleural cavity go through several important modifications which alter their physiological behavior.
With the term “post-operative residual pleural space” we refer to a free volume resulting from a pulmonary resection, which is usually diagnosed in 90% of cases of lobectomy. The residual space depends on both the pre-surgery pulmonary compliance and the pleural lymphatic vessels absorption pressure; it is usually occupied by a shift of the mediastinum and the diaphragm, by an increase in the volume of the remaining lung, and, in part, it is filled by the pleural fluid (38).
Therefore, as mentioned before, there are different mechanisms underlying the definitive lung volume.
First of all, it depends on the persistence and size of the air leak, regardless of the efficiency of the intrapleural drainage system. In fact, air flowing through the split provides a continuous refueling of the pleural space and decreases the negative intrapleural pressure. This mechanism opposes to the natural lung recoil and leads to a decrease in post-surgical lung volume.
When there is not any impairment of the lymphatic vessels drainage capacity during the pre-surgery conditions, the lymphatic flow is able to increase, minimizing the volume of fluid in the pleural cavity. On the contrary, if an alteration of the lymphatic system occurs, both pre-existed or caused by the surgery itself, this process is slowed down to the detriment of residual lung volume.
The disease that required surgery may play a significant role in the formation of the residual space. It has been demonstrated that patients treated with partial resection because of chronic pleuro-parenchymal inflammatory or infective diseases (tuberculosis, fungal infections, abscess, bronchiectasis and empyema) present a residual air space more often than patients undergoing surgery to treat lung cancers (39,40).
Another pivotal element which affects the residual lung volume is the persistence of the pre-surgery disease, such as emphysema or fibrosis. In a fibrotic lung, after pulmonary resection, pleural pressure (Pliq) becomes more sub-atmospheric and overcomes the lymphatic draining activity; this leads to an enhanced hydrothorax risk, due to the persistence of the gas bubble. On the contrary, the emphysematous lung increases Pliq implying a higher risk of air leak. Furthermore, the emphysematous tissue tends to reduce the elastic recoil while fibrosis may narrow parenchymal re-expansion due to fibrotic scares and residual granulomas (33).
Finally, the type of resection can influence the mechanism of persistent air spaces. The air leak tends to be more important and persistent after more complex and invasive operations. Technical and physical obstacles and supplement procedures, during surgery, could increase the risk of air leak. Decortication may be a compelling cause of a persisting space. The incidence of an air leak enduring longer than 5 days is about 3.5% after wedge resection, 6.7% after segmentectomy and 8.6% after lobectomy (37).
Thus, the resulting volume is the consequence of both the new equilibrium between filtration and absorption of the pleural fluid and the new mechanical coupling between the lung and the chest wall.
From the point of view of pleural space, it may be obliterated by several mechanisms.
As stated before, the remaining lung tissue struggles to expand and to fill the enlarged space. Because of the increased negative intrapleural pressure the mediastinum tends to shift towards the resection side, the diaphragm usually lifts up and the ribs move closer to each other. The consequence of these modifications is that the ribcage becomes smaller.
Furthermore, as a result of the surgery, the pleural sheets can be occupied by fibrosis or empyema or a visceral inflammatory peel. Obviously, all of these alterations tend to face the lung expansion.
As a result, the final pleural cavity depends on the balance of all of these mechanisms (39,40).
Physiology of the remaining lung
According to the definition of the respiratory system compliance (Crs = ∆V/∆P), the air volume (∆V) is equal to the ratio between compliance (Crs) and pressure (∆P): ∆V = Crs/∆P. The clearance value is calculated through the lung compliance (Cl) and the chest wall clearance (Cw) values, following the formula:
The lung and the chest wall compliances usually match and are approximately 0.2 L/cmH2O. So, the respiratory system compliance is physiologically 0.1 multiplied the lung compliance.
Considering the physiology of the lung after surgery, the compliance is strictly connected to the volume of resection. The more tissue is surgically removed the more the lung compliance is reduced. This means that in order to re-expand the lung to its original volume and fill the whole chest volume, a greater pressure is required. This over-distension may be reached to the detriment of lung natural shape. Since it is important to avoid over-distension and prevent damage of the residual lung, the post-operative pleural pressure throughout the pleural space should not exceed the pre-operative one.
This condition is improved leaving an air bubble inside the pleural cavity and regulating the pressure created by the suction line. The air bubble reduces the pleural pressure and opposes to the excessive deformation of the parenchyma. Moreover, the suction line should generate a pressure as equal as possible to the pre-operative one.
The variability of air volume is estimated monitoring the values of air bubble pressure, through a chest tube (∆V = Crs/∆P). The air pressure must be regulated in order to keep the ratio between the respiratory system and the lung compliance equal to 0.1.
According to the gradient of gas partial pressure between blood and air, the air bubble tends to be progressively reabsorbed if no leaks are present (≈1%/day). This process starts slowly, because the CO2 flow to the bubble amperes the O2 flow to the blood. Moreover, the N2 low solubility in the blood concurs to further decrease the reabsorption process. A possible approach to increase the reabsorption process could be washing the cavity with oxygen.
Another interesting aspect is that the air bubble causes a decrease in pleural pressure. According to Starling’s law this modification increases pleural fluid filtration. So pleural effusion will slowly take the place of the air bubble (38,41).
Pathological complications
When the air bubble is completely reabsorbed without being substituted by pleural fluid, over distension of the lung may occur. It usually happens at the end of inspiration, while during the expiration phase the parenchyma collapses protecting the tissue from this injure. It takes place more frequently after pneumonectomy for treating emphysema. In fact, the recoil pressure of the lung of these patients is already strongly decreased before the treatment.
Over distension is one of the most feared complications of lung resection, since it may not only produce lung injure, but it could also cause three major complications: air leak, hydrothorax and lung edema.
One of the most common problems of lung resection is the persistent air leak (PAL). Usually it is generated because of a lack in the intra-operative seal. It can be supplied by a major airway (broncho-pleural fistula) or a peripheral airway (broncho-alveolar-pleural fistula).
As well as the risk of over distension, the probability of air leak occurrence during inspiration is greater in patients treated for emphysema. This risk is also elevated in patients attending surgery to remove fibrotic tissue. As stated above, this may happen because the pressure generated inside the pleura is more negative (33).
In recent studies, Refai and coworkers have demonstrated that the duration of PAL was linked with two parameters in the immediate post-operative period: air leak flow and pleural pressure.
Even if the pleural pressure seems to be one of the most important parameters to be considered, it is not possible to monitor it closely because of the lack of a specific system. Thus, the purpose of the study was to measure, in non complicated patients, the parameters of pleural pressure with a pressure sensor.
Authors’ hypothesis was that the value of pleural pressure analyzed immediately before the removal of chest tube after non-complicated pulmonary lobectomy may be considered a “physiologic” pressure occurring after surgery during the phase of recovering. Refai and coworkers concluded that what is commonly defined as water seal (or no suction) actually expresses the pleural pressures ranging from −13 to −20 cmH2O, depending on different techniques of surgery, being aware that a large variability within the same type of lobectomy can occur (36).
Another possible complication after thoracic surgery is hydrothorax, a clinical condition characterized by a pathological liquid collection in the pleural space. The cause, however, has not to be referred only to the surgical damage but also to the proper management of the sub-atmospheric pressure applied to the chest tube.
As well known, in physiological conditions the Pliq pressure in the costo-diaphragmatic sinus is close to 0 cmH2O. After the surgical procedure, this value can change becoming positive, because of the increasing amount of flowing liquid.
It is possible to overcome the problem by placing the chest tube open to atmosphere: in this way the fluid will drain into the tube when the pressure in the hydrothorax exceeds the atmospheric pressure. In order to prevent the liquid/air draw back during inspiration, when the pressure became more negative, it could be useful to insert a one-way valve in the tube (3).
One of the most common and severe complications of thoracic surgery is lung edema.
The pathophysiological meaning can be described as an abnormal accumulation of fluid in the extravascular compartments of the lung.
Lung edema can be classified in four main categories:
- Increased hydrostatic pressure edema:
- Two pathophysiological and radiological phases are recognized in the development of pressure edema:
- Interstitial edema;
- Alveolar flooding or alveolar edema.
- These phases are virtually identical for left-sided heart failure and fluid overload.
- Two pathophysiological and radiological phases are recognized in the development of pressure edema:
- Permeability edema with diffuse alveolar damage (DAD);
- Permeability edema without diffuse alveolar damage;
- Mixed edema due to simultaneous increased hydrostatic pressure and permeability changes.
After thoracic surgery edema can be due to:
- Massive presence of fragments, reactive oxygen species and activated macrophages and neutrophils may cause alveolar insult. This damage determines inhibition of the fluid reabsorption;
- Excessive fluid perfusion volume, which overdoes the drainage capacity of the remaining pulmonary lymphatic tissue;
- Reduction of the pulmonary capillary bed with edema due to low outflow;
- Hyperinflation of the remaining lung. It has been demonstrated that avoidance of post-pneumonectomy pulmonary edema can be achieved by solely changing the management of the pneumonectomy space, through a balanced drainage system;
- As well known, local hypoxia is one of the most important factors in edema evolution. It can be caused by edema itself or by ventilation/perfusion mismatch;
- Mechanical stress on the capillaries, high intraoperative ventilation pressures, transfusions of fresh frozen plasma and abnormal pre-operative lung function increase the permeability of the alveolo-capillary membrane;
- Pulmonary hypertension and increased pulmonary vascular resistance (38,42);
- Pulmonary congestion and over distension that leads to increased microvascular filtration (33).
Future of management of pleural space after pulmonary resection
While the pre-operative assessment seems to be important to predict long-term clinical conditions, it does not permit a prediction in the early post-operative period. For this reason, an intensive early assessment may be useful to diagnose possible post-surgery initial complications. It may be of crucial importance to know the elastic properties of the lung parenchyma in order to set an appropriate pleural pressure which may prevent over distention (33).
Brunelli et al. found that the air leak flow and the pleural effusion analyzed at the sixth post-operative hour may predict the duration of the air leak (43).
The edema formation may decrease surfactant activity and cause pulmonary atelectasis. The value of instillation of surfactant is still under investigation (33).
Acknowledgments
The authors acknowledge Dr. Mattia Ramaccia for all the help during the manuscript preparation.
Funding: None.
Footnote
Provenance and Peer Review: This article was commissioned by the Guest Editors (Marco Anile, Daniele Diso) for the series “Management of Air Leaks After Thoracic Surgery” published in Journal of Xiangya Medicine. The article has undergone external peer review.
Conflicts of Interest: All authors have completed the ICMJE uniform disclosure form (available at http://dx.doi.org/10.21037/jxym.2018.03.01). The series “Management of Air Leaks After Thoracic Surgery” was commissioned by the editorial office without any funding or sponsorship. The authors have no other conflicts of interest to declare.
Ethical Statement: The authors are accountable for all aspects of the work in ensuring that questions related to the accuracy or integrity of any part of the work are appropriately investigated and resolved.
Open Access Statement: This is an Open Access article distributed in accordance with the Creative Commons Attribution-NonCommercial-NoDerivs 4.0 International License (CC BY-NC-ND 4.0), which permits the non-commercial replication and distribution of the article with the strict proviso that no changes or edits are made and the original work is properly cited (including links to both the formal publication through the relevant DOI and the license). See: https://creativecommons.org/licenses/by-nc-nd/4.0/.
References
- Miserocchi G, Agostoni E. Contents of the pleural space. J Appl Physiol 1971;30:208-13. [Crossref] [PubMed]
- Negrini D. Integration of capillary, interstitial and lymphatic function in the pleural space. In: Reed RK, McHale NG, Bert JL, et al. editors. Interstitium Connective Tissues and Lymphatics. London: Portland Press, 1995:283-99.
- Belvisi MG. Overview of the innervation of the lung. Curr Opin Pharmacol 2002;2:211-5. [Crossref] [PubMed]
- Standring S. editor. Gray’s Anatomy, the anatomical basis of clinical practice. 41st edition. Elsevier Science, 2009:989-92.
- Gibson J, Geddes D, Costabel U, et al. Respiratory Medicine. 3rd edition. Saunders Ltd, 2003:34-50.
- Hills BA. Graphite-like lubrication of mesothelium by oligolamellar pleural surfactant. J Appl Physiol 1992;73:1034-9. [Crossref] [PubMed]
- Owens MW, Milligan SA, Grisham MB. Nitric oxide synthesis by rat pleural mesothelial cells: induction by growth factors and lipopolysaccharide. Exp Lung Res 1995;21:731-42. [Crossref] [PubMed]
- Owens MW, Grisham MB. Nitric oxide synthesis by rat pleural mesothelial cells: induction by cytokines and lipopolysaccharide. Am J Physiol 1993;265:L110-6. [PubMed]
- Heine H, Ulmer AJ. Recognition of bacterial products by toll-like receptors. Chem Immunol Allergy 2005;86:99-119. [Crossref] [PubMed]
- Delves PJ, Roitt IM. The immune system: second of two parts. N Engl J Med 2000;343:108-17. [Crossref] [PubMed]
- Thomas R, Cheah HM, Creaney J, et al. Longitudinal Measurement of Pleural Fluid Biochemistry and Cytokines in Malignant Pleural Effusions. Chest 2016;149:1494-500. [Crossref] [PubMed]
- Jantz MA, Antony VB. Pathophysiology of the pleura. Respiration 2008;75:121-33. [Crossref] [PubMed]
- Zocchi L. Physiology and pahophysiology of pleural fluid turnover. Eur Respir J 2002;20:1545-58. [Crossref] [PubMed]
- Shidham V, Atkinson B. Cytopathologic Diagnosis of Serous Fluids. 1st edition. Philadelphia, Pennsylvania: Saunders Elsevier, 2007.
- Lee YC, Light RW. Pleural effusion: overview. In: Laurent G, Shapiro S. editors. Encyclopedia of respiratory diseases, 4th edition. Oxford, UK: Elsevier, 2006:353-8.
- Guyton AC, Hall JE. Textbook of Medical Physiology. 12th edition. Saunders Elsevier, 2011:483-4.
- Miserocchi G, Negrini D. Pleural space: pressure and fluid dynamics. In: Crystal RG, West JB. editors. The lung, Scientific Foundations. New York: Raven Press, 1997:1217-25.
- Miserocchi G, Venturoli D, Negrini D, et al. Model of pleural fluid turnover. J Appl Physiol 1993;75:1798-806. [Crossref] [PubMed]
- Miserocchi G. Mechanisms controlling the volume of pleural liquid and extravascular lung water. Eur Respir Rev 2009;18:244-52. [Crossref] [PubMed]
- Brunelli A, Beretta E, Cassivi SD, et al. Consensus definitions to promote an evidence-based approach to management of the pleural space. A collaborative proposal by ESTS, AATS, STS, and GTSC. Eur J Cardiothorac Surg 2011;40:291-7. [Crossref] [PubMed]
- Butler JP, Huang J, Loring SH, et al. Model for a pump that drives circulation of pleural fluid. J Appl Physiol (1985) 1995;78:23-9. [PubMed]
- Miserocchi G, Nakamura T, Mariani E, et al. Pleural liquid pressure over the interlobar mediastinal and diaphragmatic surfaces of the lung. Respir Physiol 1981;46:61-9. [Crossref] [PubMed]
- Miserocchi G, Venturoli D, Negrini D, et al. Intrapleural fluid movements described by a porous flow model. J Appl Physiol 1992;73:2511-6. [Crossref] [PubMed]
- Smyrnios NA, Jederlinic PJ, Irwin RS. Pleural effusion in an asymptomatic patient: spectrum and frequency of causes and management considerations. Chest 1990;97:192-6. [Crossref] [PubMed]
- Light RV. Disorders of the pleura. In: Harrison’s Principles of Internal medicine. 19th edition. McGraw-Hill Education, 2015:1716-19.
- Culotta R, Tylor D. Diseases of the pleura. In: Ali J, Summer WR, Levitzky MG. editors. Pulmonary pathophysiology. 2nd edition. New York: MgGraw-Hill, 2005:194-212.
- Wiener-Kronish JP, Matthay MA. Pleural effusions associated with hydrostatic and increased permeability pulmonary edema. Chest 1988;93:852-8. [Crossref] [PubMed]
- Eid AA, Keddissi JI, Kinasewitz GT. Hypoalbuminemia as a cause of pleural effusions. Chest 1999;115:1066-9. [Crossref] [PubMed]
- Miserocchi G. Physiology and pathophysiology of pleural fluid turnover. Eur Respir J 1997;10:219-25. [Crossref] [PubMed]
- Hyde L. Benign spontaneous pneumothorax. Ann Intern Med 1962;56:746-51. [Crossref] [PubMed]
- Greene R, McLoud TC, Stark P. Pneumothorax. Semin Roentgenol 1977;12:313-25. [Crossref] [PubMed]
- Antoni RO, Ponka JL. The hazards of iatrogenic pneumothorax in certain diagnostic and therapeutic procedures. Surg Gynecol Obstet 1961;113:24-32. [PubMed]
- Miserocchi G, Beretta E, Rivolta I. Respiratory mechanics and fluid dynamics after lung resection surgery. Thorac Surg Clin 2010;20:345-57. [Crossref] [PubMed]
- Haber R, Grotberg JB, Glucksberg MR, et al. Steady - state pleural fluid flow and pressure and the effects on the lung buoyancy. J Biomech Eng 2001;123:485-92. [Crossref] [PubMed]
- Miserocchi G, Negrini D, Pistolesi M, et al. Intrapleural liquid flow down a gravity-dependent hydraulic pressure gradient. J Appl Physiol (1985) 1988;64:577-84. [PubMed]
- Refai M, Brunelli A, Varela G, et al. The values of intrapleural pressure before the removal of chest tube in non-complicated pulmonary lobectomies. Eur J Cardiothorac Surg 2012;41:831-3. [Crossref] [PubMed]
- Pompili C, Miserocchi G. Air leak after lung resection: pathophysiology and patients' implications. J Thorac Dis 2016;8:S46-54. [PubMed]
- Miserocchi G, Beretta E. Pathophysiology of extravascular water in the pleural cavity and in the lung interstitium after lung thoracic surgery. In: Guerreiro Cardoso PF. editor. Topics in Thoracic Surgery. 2012;25:434-43.
- Barker WL. Natural history of residual air spaces after pulmonary resection. Chest Surg Clin N Am 1996;6:585-613. [PubMed]
- Misthos P, Kokotsakis J, Konstantinou M, et al. Postoperative residual pleural spaces: characteristics and natural history. Asian Cardiovasc Thorac Ann 2007;15:54-8. [Crossref] [PubMed]
- Varela G, Jiménez MF, Novoa NM, et al. Postoperative chest tube management: measuring air leak using an electronic device decreases variability in the clinical practice. Eur J Cardiothorac Surg 2009;35:28-31. [Crossref] [PubMed]
- Sengupta S. Post-operative pulmonary complications after thoracotomy. Indian J Anaesth 2015;59:618-26. [Crossref] [PubMed]
- Brunelli A, Cassivi SD, Salati M, et al. Digital measurements of air leak flow and intrapleural pressure in the immediate post-operative period predict risk of prolonged air leak after pulmonary lobectomy. Eur J Cardiothorac Surg 2011;39:584-8. [Crossref] [PubMed]
Cite this article as: Paone G, De Rose G, Giudice GC, Cappelli S. Physiology of pleural space after pulmonary resection. J Xiangya Med 2018;3:10.