Hepatocyte growth factor (HGF) and insulin-like growth factor 1 (IGF-1) improved ventricular remodeling after myocardial infarction through inhibiting MMP8/13 in a rat model
Introduction
Myocardial infarction (MI) is a severe type of coronary artery disease. With the activation of local inflammation and the stimulation of cytokines, the heart changes at the cellular and extracellular matrix (ECM) level, leading to ventricular remodeling (VR), eventually cause heart failure (1,2).
Beta blocker and angiotensin-converting enzyme inhibitor/angiotensin receptor blocker (ACEI/ARB) have been proved in improving VR and prognosis after MI, but they cannot completely prevent the occurrence of heart failure (3,4). In the last decade, stem cell therapy has become a hot spot in basic and clinical research (1-6). However, there are different conclusions about the effectiveness. Moreover, the potent to arrhythmia partly affect its clinical trials (7-10). It has been suggested that the potential benefits of stem cell therapy after MI may derive from a variety of cytokines secreted by the cells, including hepatocyte growth factor (HGF) and insulin-like growth factor 1 (IGF-1) (11,12). HGF and IGF-1 has been reported on promoting the recovery after MI (13-15). But whether combination of HGF and IGF-1 enhances the effect and how it works is still not fully understood.
In the process of myocardial fibrosis, matrix metalloproteinase (MMPs) play an important role (16). MMPs are a series of zinc ions dependency endogenous enzymes, which degrade the ECM components mainly (17). It has been reported that on the edge of infarcted area, the increasing expression of MMP8/13 had been observed, which indicates it may be related to VR process after MI. But the mechanism stayed unknown (18).
The application of MI SD rat model is an important means to study the scientific problems related to MI, and the conclusions come from which may have a good relevance to human biology. We present the following article in accordance with the ARRIVE reporting checklist (available at http://dx.doi.org/10.21037/jxym-20-95).
Objectives
Here we try to figure out how combination therapy of HGF and IGF-1 influence the expression of MMP8/13 after MI and what the possible mechanism may be.
Methods
Preparation for rat myocardial infarction model
Forty-eight male SD rats weighing 300–350 g were fasted all night except for free access to water. Then the rats were anesthetized with pentobarbital (concentration: 1%, 0.4–0.5 mL/100 g) by intraperitoneal injection. Endotracheal intubation was performed under a 14-gauge cannula mounted on a blunt and hollow needle with a 145° angled tip. The rats were mechanically ventilated with room air at a tidal volume of 0.5 mL/100 g body weight and a frequency of 100 breaths/min. The electrocardiogram Lead II was selected for continuous monitoring (BIOPAC MP150). A thoracotomy was performed via the left fourth intercostal space. Then, the pericardium was incised, and the left atrial appendage was elevated to expose the left anterior descending coronary artery. The left anterior descending coronary artery was ligated 2 mm below the left atrial appendage by using a 5/0 nylon suture. Successful occlusion was confirmed electrocardiographically by ST segment elevation. Drainage tubes were used to withdraw air or blood in the cavity while the chest is closed. The rats recovered from anesthesia and were then placed back into their cages for 2 weeks. Intramuscular injection of penicillin was administered post-operatively for prevention of infection.
Injection of cytokine solution
Two weeks after surgical intervention, the 34 surviving rats were anesthetized and orally intubated as described above. The rats were mechanically ventilated with room air, and a new thoracotomy was performed. The animals were randomized to receive phosphate buffer solution (PBS group, n=8), HGF solution (HGF group, concentration: 100 ng/mL, n=9), IGF-1 solution (IGF-1 group, concentration: 150 ng/mL, n=9) and a mix of HGF and IGF-1 (GFs group, concentration: HGF:100 ng/mL, IGF-1:150 ng/mL, n=8) by injection into the border of infarcted area. Successful injection was confirmed by the formation of a bled covering the infarcted zone. After recovering from anesthesia, the rats were placed back into their cages for 6 weeks. Prevention of postoperative infection was controlled as described above.
Ultrasonic cardiogram detection
Two weeks after the surgical intervention and 6 weeks after the PBS or cytokine solution injection, we performed ultrasonic cardiogram under 1% pentobarbital anesthesia (0.4–0.5 mL/100 g body weight) to detect the cardiac structure and function including left ventricular diastolic diameter (LVIDd), left ventricular systolic diameter (LVIDs), interventricular septum (IVS), left ventricular posterior wall (LVPW), left ventricular ejection fraction (LVEF) and left ventricular fractional shortening (LVFS). Then, the difference of each index between before and after cytokine injection treatment was assessed (dLVIDd, dLVIDs, dIVS, dLVPW, dLVEF, dLVFS).
Tissue collection
After ultrasonic examination, the rats were killed by cervical dislocation and hearts were harvested. The left ventricular myocardium was cut in half through the center of the infarcted area. One half was immediately stored in −80 °C for further processing, and the other half for histopathological examination of hematoxylin and eosin (HE) staining and Masson staining. The infarcted region was visually identified by a mottled and pale discoloration. The border zone was myocardium which extended 1.0–2.0 mm from the infarcted area.
Western blotting analysis
Frozen border-zone samples were analyzed by Western blotting using the specific antibodies against MMP8 (1:1,000, Invitrogen, USA), MMP13 (1:1,000, Invitrogen, USA), p38 mitogen activated protein kinase (p38 MAPK, 1:1,000, Invitrogen, USA) and phosphatidyl inositol 3-kinase (PI3K, 1:1,000, Invitrogen, USA). To ensure equal loading, the same membranes were probed with GAPDH. Bands were visualized by enhanced chemiluminescence and quantified by the Quantity One Software (BioRad).
Real-time quantitative reverse transcription polymerase chain reaction (qRT-PCR)
Real-time qRT-PCR was performed according to the manufacturer’s protocol. Total RNA was extracted from the border-zone with TRIzol reagent (Invitrogen, USA), and reversely transcribed to cDNA using M-MLV Reverse Transcriptase (Promega, USA). qRT-PCR was performed to measure the expression levels of candidate genes using a SYBR Green PCR Master mix (Toyobo, Japan). As an internal control, the expression of ACTB mRNA was estimated with the SYBR green system. The specific primer sequences of the selected genes are listed in Table 1.
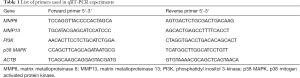
Full table
HE staining
Samples taken from the border zone were routinely processed. Three sections of each heart were used for HE staining study. We examined the morphology of the myocardial cell, the inflammatory infiltration and the arrangement of cardiac muscle fibers. Each slide was examined under a microscope (Axio Scope A1, Zeiss).
Masson staining
Samples taken from the border zone were routinely processed. Three sections of each heart were used for Masson staining study. The fibrous tissue was stained blue. The area of stained fiber was determined by Image-Pro Plus 6.0 analysis system (Media Cybemetics, USA). Each slide was examined under a microscope (Axio Scope A1, Zeiss) and fibrous area was calculated by scoring 5 randomly-selected microscopic fields.
Statistical analysis
All quantitative data was described as mean ± standard deviation (SD). If data met normal distribution and homogeneity of variance, one-way ANOVA test was performed in comparisons between groups. Otherwise, we chose Kruskal-Wallis instead. A value of P<0.05 was consider statistically significant. The tests were performed with SPSS 20.0 software.
Ethical statement
The study was conducted in accordance with the Declaration of Helsinki (as revised in 2013). All experimental procedures were performed under licensed by the Animal Ethics Committee of the Fifth Affiliated Hospital of Sun Yat-sen University, in compliance with National Research Council guidelines for the care and use of animals (publication No. 85-23 revised 2011). No consent was required from patients since no patients were involved in this study.
Results
Six weeks after the PBS or cytokine solution injection, all surviving rats in each group {PBS group [6/8], HGF group [5/9], IGF-1 group [6/9] and GFs group [6/8]} were included in the statistical analysis.
GFs improved cardiac structure and function
Compared with PBS group, HGF, IGF-1 and GFs group indicated improving cardiac structure and function with dLVIDd, dLVIDs and dIVS decreased while the dLVEF and dLVFS increased (P<0.05, respectively). Besides, the decrease of dLVIDd and dLVIDs in GFs group was more significant compared with HGF group and IGF-1 group (P<0.05, respectively) (Table 2 and Figure 1).
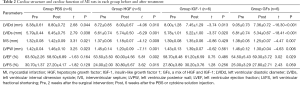
Full table
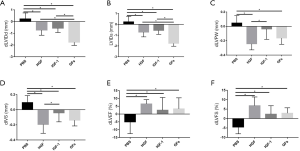
GFs decreased inflammatory infiltration and reduced infarcted area
The myocardial cells in the PBS group were swollen and disordered, with numerous infiltrating inflammatory cells. In HGF, IGF-1 and GFs group, myocardial cell arranged regularly, cytoplasm swelled lighter and inflammatory cell infiltration decreased compared with PBS group. The effect was more obvious in GFs group (Figure 2A,B,C,D).
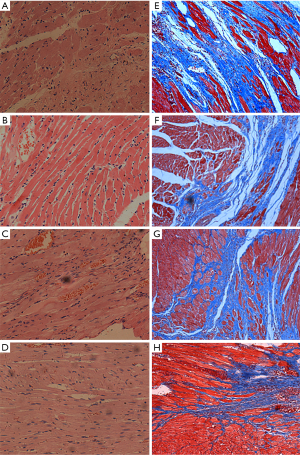
Typical Masson staining results of each group were shown in Figure 2E,F,G,H. It can be seen that the infarcted myocardial tissue was stained light blue because collagen replaced normal myocardial cells, and the remaining normal myocardial tissue was stained red. In PBS group, the infarcted area ventricular wall thinned and left ventricular space expanded. Compared with PBS group, the area of myocardial fibrosis decreased in HGF and IGF-1 group, which was more obvious in GFs group (Figure 2E,F,G,H).
GFs reduced the expression of MMP8/13 and increased p38 MAPK and PI3K
In HGF, IGF-1 and GFs group, mRNA and protein expression of MMP8/13 significantly decreased on the border-zone as compared with those in PBS group (P<0.05, respectively). And GFs group further decreased protein expression of MMP8/13 compared with HGF and IGF-1 group while no significant difference was seen between HGF and GFs group in mRNA level (P>0.05). Compared with PBS group, the mRNA and protein expression of p38 MAPK in GFs and HGF group increased (P<0.05, respectively) and no significant difference was seen between HGF and GFs group (P>0.05, respectively). Similarly, compared with PBS group, the mRNA and protein expression of PI3K in GFs and IGF-1 group increased (P<0.05, respectively) and no significant difference was seen between them (P>0.05, respectively) (Figures 3,4).
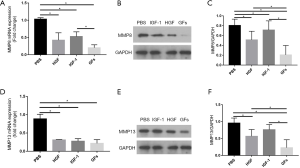
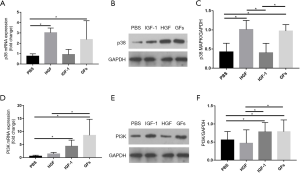
Discussion
The cardiac protective effects of HGF and IGF-1 have been greatly reported. They can improve cardiac structure and function through promoting angiogenesis and activating cardiac progenitor cells after MI (19-23). But what is the role about HGF and IGF-1 act on the ECM and weather HGF combined with IGF-1 have a synergistic effect are not fully understood. In our experiments, combination therapy of HGF and IGF-1 after MI reduced the fiber deposition on the border zone and improved the cardiac function through suppressing the expression of MMP8/13, and the effect may be more remarkable than any single use to some extent.
Collagen is the main component of ECM in heart, among which, type I/III account for up to 90%. They play an important role in maintaining constitutional and functional integrity of heart. Collagen metabolism will be active after MI. In the infarcted zone, collagen degrade with myocytes necrosis, while on the border zone, collagen fibers deposit abnormally, leading to structure network destroy and making cardiac function decrease (24-26).
Decomposition of collagen is modified by MMPs family, a series of zinc irons which mainly participate in the metabolic activity of ECM. MMP8/13 can degrade type I/III collagen specifically (27). It has been reported that expression of MMP8/13 greatly increased on border zone after MI, prompting that it may have some pathologic significance in the remodeling process (28). In this study, we found that HGF and IGF-1 could both decrease the degree of fibrosis and improve myocardial fiber alignment on the border zone accompanied by the expression of MMP8/13 inhibited.
It is reported that MMPs can be regulated by various signal pathways, including MAPK and PI3K/Akt (29-31). MAPK is a highly conservative group of serine/threonine kinases, which plays an important role in the signal transduction process. As a classical pathway of MAPK, p38 MAPK involves in cellular activities of activation, migration and differentiation of HGF (32,33). PI3K consists of p85 and p110 subunits with the activity of serine/threonine kinase and phosphatidyl inositol kinase. It can cause cascade activation of Akt to participate in the cells of proliferation, differentiation and apoptosis. Studies have shown that IGF-1 can increase the expression of effector molecules, such as Bcl-2, α-actin, IGF and connexin-5 to inhibit cell apoptosis, increase myocardial contractility and modify the collagen synthesis activity of fibroblasts (34). In this study, we found that HGF could increase the expression of p38 MAPK while IGF-1 increased the expression of PI3K/Akt. It indicated that HGF and IGF-1 may play a role in inhibiting VR through different pathways.
Conclusions
HGF and IGF-1 improve VR after MI through suppressing the expression of MMP8/13 on the border zone. It suggests that combination therapy of HGF and IGF-1 can play an even greater role than any single treatment to some extent. HGF and IGF-1 may suppress the expression of MMP8/13 through p38 MAPK and PI3K pathway respectively.
Acknowledgments
Funding: This work was supported by the Science and Technology Planning Project of Guangdong province (grant No. 2014A020212088). The Major Program of Science and Technology Planning Project in Medical and Health of Zhuhai (grant No. 2015B1031).
Footnote
Reporting Checklist: The authors have completed the ARRIVE reporting checklist. Available at http://dx.doi.org/10.21037/jxym-20-95.
Conflicts of Interest: All authors have completed the ICMJE uniform disclosure form (available at http://dx.doi.org/10.21037/jxym-20-95). All authors report grants from Science and Technology Planning Project of Guangdong province, grants from The Major Program of Science and Technology Planning Project in Medical and Health of Zhuhai, during the conduct of the study. The authors have no other conflicts of interest to declare.
Ethical Statement: The authors are accountable for all aspects of the work in ensuring that questions related to the accuracy or integrity of any part of the work are appropriately investigated and resolved. The study was conducted in accordance with the Declaration of Helsinki (as revised in 2013). All experimental procedures were performed under licensed by the Animal Ethics Committee of the Fifth Affiliated Hospital of Sun Yat-sen University, in compliance with National Research Council guidelines for the care and use of animals (publication No. 85-23 revised 2011). No consent was required from patients since no patients were involved in this study.
Open Access Statement: This is an Open Access article distributed in accordance with the Creative Commons Attribution-NonCommercial-NoDerivs 4.0 International License (CC BY-NC-ND 4.0), which permits the non-commercial replication and distribution of the article with the strict proviso that no changes or edits are made and the original work is properly cited (including links to both the formal publication through the relevant DOI and the license). See: https://creativecommons.org/licenses/by-nc-nd/4.0/.
References
- Rota M, Padin-Iruegas ME, Misao Y, et al. Local activation or implantation of cardiac progenitor cells rescues scarred infarcted myocardium improving cardiac function. Circ Res 2008;103:107-16. [Crossref] [PubMed]
- Cohn JN, Ferrari R, Sharpe N. Cardiac remodeling--concepts and clinical implications: a consensus paper from an international forum on cardiac remodeling. behalf of an international forum on cardiac remodeling. J Am Coll Cardiol 2000;35:569-82. [Crossref] [PubMed]
- Choi D, Hwang KC, Lee KY, et al. Ischemic heart diseases: current treatments and future. J Control Release 2009;140:194-202. [Crossref] [PubMed]
- White HD, Chew DP. Acute myocardial infarction. Lancet 2008;372:570-84. [Crossref] [PubMed]
- Duran JM, Makarewich CA, Sharp TE, et al. Bone-derived stem cells repair the heart after myocardial infarction through transdifferentiation and paracrine signaling mechanisms. Circ Res 2013;113:539-52. [Crossref] [PubMed]
- Karantalis V, Hare JM. Use of mesenchymal stem cells for therapy of cardiac disease. Circ Res 2015;116:1413-30. [Crossref] [PubMed]
- Lipinski MJ, Biondi-Zoccai GG, Abbate A, et al. Impact of intracoronary cell therapy on left ventricular function in the setting of acute myocardial infarction: a collaborative systematic review and meta-analysis of controlled clinical trials. J Am Coll Cardiol 2007;50:1761-7. [Crossref] [PubMed]
- Macia E, Boyden PA. Stem cell therapy is proarrhythmic. Circulation 2009;119:1814-23. [Crossref] [PubMed]
- Henning RJ. Stem cells for cardiac repair: problems and possibilities. Future Cardiol 2013;9:875-84. [Crossref] [PubMed]
- Gómez-Mauricio G, Moscoso I, Martín-Cancho MF, et al. Combined administration of mesenchymal stem cells overexpressing IGF-1 and HGF enhances neovascularization but moderately improves cardiac regeneration in a porcine model. Stem Cell Res Ther 2016;7:94. [Crossref] [PubMed]
- Bagno LL, Carvalho D, Mesquita F, et al. Sustained IGF-1 secretion by adipose-derived stem cells improves infarcted heart function. Cell Transplant 2016;25:1609-22. [Crossref] [PubMed]
- Ellison GM, Torella D, Dellegrottaglie S, et al. Endogenous cardiac stem cell activation by insulin-like growth factor-1/hepatocyte growth factor intracoronary injection fosters survival and regeneration of the infarcted pig heart. J Am Coll Cardiol 2011;58:977-86. [Crossref] [PubMed]
- Nakamura T, Sakai K, Nakamura T, et al. Hepatocyte growth factor twenty years on: much more than a growth factor. J Gastroenterol Hepatol 2011;26:188-202. [Crossref] [PubMed]
- Azuma J, Taniyama Y, Takeya Y, et al. Angiogenic and antifibrotic actions of hepatocyte growth factor improve cardiac dysfunction in porcine ischemic cardiomyopathy. Gene Ther 2006;13:1206-13. [Crossref] [PubMed]
- Phatharajaree W, Phrommintikul A, Chattipakorn N. Matrix metalloproteinases and myocardial infarction. Can J Cardiol 2007;23:727-33. [Crossref] [PubMed]
- Creemers EE, Cleutjens JP, Smits JF, et al. Matrix metalloproteinase inhibition after myocardial infarction: A new approach to prevent heart failure? Circ Res 2001;89:201-10. [Crossref] [PubMed]
- Fan D, Takawale A, Lee J, et al. Cardiac fibroblasts, fibrosis and extracellular matrix remodeling in heart disease. Fibrogenesis Tissue Repair 2012;5:15. [Crossref] [PubMed]
- Troncoso R, Ibarra C, Vicencio JM, et al. New insights into Igf-1 signaling in the heart. Trends Endocrinol Metab 2014;25:128-37. [Crossref] [PubMed]
- Ren J, Samson WK, Sowers JR. Insulin-like growth factor I as a cardiac hormone: Physiological and pathophysiological implications in heart disease. J Mol Cell Cardiol 1999;31:2049-61. [Crossref] [PubMed]
- Gallo S, Sala V, Gatti S, et al. Cellular and molecular mechanisms of HGF/Met in the cardiovascular system. Clin Sci (Lond) 2015;129:1173-93. [Crossref] [PubMed]
- Liu J, Wu P, Wang Y, et al. Ad-HGF improves the cardiac remodeling of rat following myocardial infarction by upregulating autophagy and necroptosis and inhibiting apoptosis. Am J Transl Res 2016;8:4605-27. [PubMed]
- Sonnenberg SB, Rane AA, Liu CJ, et al. Delivery of an engineered HGF fragment in an extracellular matrix-derived hydrogel prevents negative LV remodeling post-myocardial infarction. Biomaterials 2015;45:56-63. [Crossref] [PubMed]
- Fedak PW, Verma S, Weisel RD, et al. Cardiac remodeling and failure from molecules to man (Part II). Cardiovasc Pathol 2005;14:49-60. [Crossref] [PubMed]
- Dixon JA, Gorman RC, Stroud RE, et al. Mesenchymal cell transplantation and myocardial remodeling after myocardial infarction. Circulation 2009;120:S220-9. [Crossref] [PubMed]
- Lu L, Chen SS, Zhang JQ, et al. Activation of nuclear factor-kappaB and its proinflammatory mediator cascade in the infarcted rat heart. Biochem Biophys Res Commun 2004;321:879-85. [Crossref] [PubMed]
- Klein T, Bischoff R. Physiology and pathophysiology of matrix metalloproteases. Amino Acids 2011;41:271-90. [Crossref] [PubMed]
- Peterson JT, Li H, Dillon L, et al. Evolution of matrix metalloprotease and tissue inhibitor expression during heart failure progression in the infarcted rat. Cardiovasc Res 2000;46:307-15. [Crossref] [PubMed]
- Lee HS, Miau LH, Chen CH, et al. Differential role of p38 in Il-1alpha induction of MMP-9 and MMP-13 in an established liver myofibroblast cell line. J Biomed Sci 2003;10:757-65. [PubMed]
- Chen D, Li Y, Dai X, et al. 1,25-Dihydroxyvitamin D3 activates MMP13 gene expression in chondrocytes through p38 MARK pathway. Int J Biol Sci 2013;9:649-55. [Crossref] [PubMed]
- Song G, Ouyang G, Bao S. The Activation of Akt/Pkb signaling pathway and cell survival. J Cell Mol Med 2005;9:59-71. [Crossref] [PubMed]
- Forte G, Minieri M, Cossa P, et al. Hepatocyte growth factor effects on mesenchymal stem cells: Proliferation, migration, and differentiation. Stem Cells 2006;24:23-33. [Crossref] [PubMed]
- Su Y, Xie W, Wang C, et al. Jnk/P38 mitogen-activated protein kinase used for hepatocyte growth factor-induced proliferation, differentiation, and migration in human dental papilla Cells. J Endod 2012;38:1207-13. [Crossref] [PubMed]
- Delaughter MC, Taffet GE, Fiorotto ML, et al. Local insulin-like growth factor I expression induces physiologic, then pathologic, cardiac hypertrophy in transgenic mice. FASEB J 1999;13:1923-9. [Crossref] [PubMed]
- McMullen JR, Shioi T, Huang WY, et al. The insulin-like growth factor 1 receptor induces physiological heart growth via the phosphoinositide 3-Kinase(P110Alpha) pathway. J Biol Chem 2004;279:4782-93. [Crossref] [PubMed]
Cite this article as: Tan G, Chen Y, Huang Y, Yao J, Huang Y, Chen J, Wu W. Hepatocyte growth factor (HGF) and insulin-like growth factor 1 (IGF-1) improved ventricular remodeling after myocardial infarction through inhibiting MMP8/13 in a rat model. J Xiangya Med 2021;6:15.