A single center case series in three-dimensional printing for preoperative planning and surgical education: applications in cardiothoracic surgery, otolaryngology, and general surgery
Introduction
Three-dimensional (3D) printing is a remarkable technology that has gained widespread acclaim in recent years. Also known as additive manufacturing, the process allows computer-based models to be transformed into real physical objects in a layer-by-layer fashion (1-3). 3D printing has sparked revolutionary advances in the industrial field and is actively being implemented in medicine, where the largest share of application exists in the surgical arena (1,4).
Among all surgical disciplines, a precise anatomical understanding is fundamental for proper planning and optimal outcomes. This knowledge is usually obtained from two-dimensional (2D) imaging studies (4), most often computed tomography (CT) and magnetic resonance imaging (MRI). While these modalities are sufficient for routine surgical cases, they often leave complex spatial relationships in more complicated cases difficult to interpret. Although 3D virtual reconstructions partially address this issue, the advent of 3D printing promises superior anatomical orientation, allowing surgeons to physically manipulate a real 3D model rather than attempting to visualize 3D structures in their minds (4). This combination of tactile and visual feedback improves comprehension of anatomical details, reduces the time required to achieve it, and more accurately mimics the operating room environment compared to conventional imaging methods (3-5).
When 3D printed models are used in the preoperative setting, each step of the procedure can be traced directly on the model, allowing the surgeon to foresee potential challenges and determine the optimal surgical approach (4). The model can also be sterilized, brought into the operating room, and placed in the operative field for reference during the procedure. In addition, recent improvements in 3D printing materials and software have allowed the recreation of human-like tissue that possesses similar structural and mechanical properties to anatomical areas of interest (4,6). Through “surgical simulation” or “virtual surgery”, surgeons can then practice the intended procedure on a 3D model exhibiting such traits (4,7). This provides exceptional opportunities for surgical trainees to practice essential procedural skills and techniques to a level not otherwise attainable amid limited work hours and strict quality standards in today’s training environment (3,7).
The emerging literature on 3D printing in surgery has grown tremendously in the last decade and demonstrated the potential to reduce surgical times, decrease radiation exposure, and improve medical outcomes (1,2,5,8). While most of the evidence is qualitative from case reports and series, early work is promising, and it remains imperative for hospital groups and field-leading experts to communicate their experiences in order to identify the most effective uses of this technology going forward. Here we report our single-center experience with 3D printed models in preoperative planning and surgical education across several surgical subspecialties including cardiothoracic surgery, otolaryngology, and general surgery. We highlight 5 patients with congenital heart disease, 2 patients with congenital airway stenosis, 1 patient with congenital vascular disease, and 1 patient with solid hepatic tumor. We present the following article in accordance with the AME Case Series reporting checklist (available at https://dx.doi.org/10.21037/jxym-21-32).
Methods
Between November 2017 and October 2019, a total of 9 patients (aged 8 months to 52 years) were selected based on the clinical need for more in-depth surgical planning with 3D printed models. For each patient, we used preoperative CT data to create 3D printed models. Of the 9 models in this case series, 8 were used for preoperative planning and 1 was used postoperatively for surgical simulation.
We produced 3D printed models through a standard 3D printing procedure involving several steps. First, we used raw CT data to build a 3D dataset. This dataset was segmented using Mimics® software (Materialise Interactive Medical Image Control System, Materialise NV, Belgium, RRID:SCR_012153). Segments were then converted into STL (STerioLithography) file format which was used to print the 3D model from a Stratasys J750 printer. For further details of the technical specification and workflow please refer to the attached supplement (Appendix 1).
Ethics statement
The study was conducted in accordance with the Declaration of Helsinki (as revised in 2013). The study did not require IRB approval and individual consent for this retrospective analysis was waived.
Results
Cardiothoracic surgery
Complex cases of congenital heart disease (CHD) present unique challenges in the perioperative setting. Often involving unpredictable anatomy and complicated by previous surgical repairs, these cases require extensive planning and deliberation and heavily rely on multimodal advanced cardiac imaging (9). But despite major advancements in today’s imaging technology, obtaining a true understanding of the spatial relationships involved is difficult to achieve from images alone. For this reason, 3D printed models have been increasingly utilized in complex CHD cases and have the potential to improve many procedural and educational outcomes (2,5,8-10). Here we present 5 cases where 3D printed models enhanced the care of patients with CHD, including 3 cases in pediatric cardiothoracic surgery, 1 case in adult cardiothoracic surgery, and 1 case in pediatric interventional cardiology.
Case 1
Case 1 is a 3-month-old female diagnosed with inferior sinus venosus atrial septal defect (ISVASD) and partial anomalous pulmonary venous return (PAPVR). She was considered for catheter-based left upper pulmonary vein balloon angioplasty and subsequent surgical repair of PAPVR and ISVASD. During preoperative planning, there was question of whether there would be inferior vena cava (IVC) narrowing and hence, if IVC reimplantation into the right atrium would be indicated. In order to assess the feasibility of this approach, a 3D model was printed and allowed for superior evaluation of the IVC and its relation to the ISVASD. (Figure 1A). Using this information, surgical repair of the ISVASD and PAPVR with IVC reimplantation into the right atrium was planned and performed without complication. Postoperatively, it was noted that without IVC reimplantation, the IVC baffle used likely would have been at risk of obstruction following the ISVASD repair. By avoiding baffle obstruction, the incorporation of the 3D model in preoperative planning ultimately made the surgeon to take a novel approach.
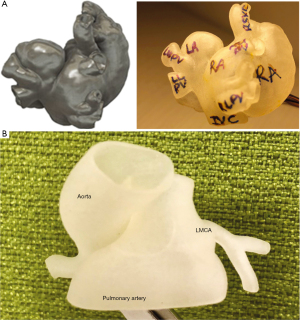
Case 2
Case 2 is a 17-month-old female with dilated cardiomyopathy secondary to anomalous left coronary artery from the pulmonary artery (ALCAPA). CT angiography revealed the ALCAPA arising from the non-facing (left anterior) pulmonary sinus of the main pulmonary artery. Given the atypical location of the anomalous left coronary artery, surgical repair was thought to require a longer baffle and be more difficult than a routine ALCAPA repair. A unique surgical approach was proposed, involving a baffle from the ALCAPA to the aorta through the pulmonary artery. Because of this, a 3D model (Figure 1B) was printed and used for preoperative planning. Subsequent surgical repair involved translocation of the ALCAPA arising from the non-facing pulmonary sinus with two-cusp excision and tunnel baffle to the ascending aorta. This was a novel way of thinking and utilizing the information for better planning.
Case 3
Case 3 is an 18-month-old female born at 30 weeks’ gestation with double outlet right ventricle (DORV), transposition of the great arteries (TGA) with severe left ventricular outflow tract obstruction, subpulmonic stenosis, and ventricular septal defect (VSD), who had previously undergone modified aortopulmonary central shunt. Optimal repair for this patient’s DORV and VSD was initially deemed to be a left ventricle to aorta baffle through the VSD. However, the CT data was inconclusive as to whether this operation would be possible. A 3D model was thus printed and used for preoperative planning. The 3D model revealed an unobstructed pathway from the left ventricle to the aorta via the VSD, hence making her a candidate for two-ventricle repair with a left ventricle to aorta baffle and right ventricle to pulmonary artery conduit. Surgical repair was performed as planned without adverse event, but postoperative course was complicated by heart block requiring pacing, rhinovirus infection, and VSD patch leak. The 3D printed model (Figure 2) helped us to be clear about the pathway from left ventricle to aorta via the VSD as seen intraoperatively.
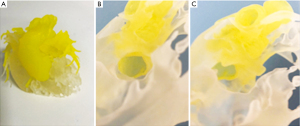
Case 4
Case 4 is a 52-year-old male with superior sinus venosus atrial septal defect (SSVASD) and PAPVR, found to have atrial fibrillation. A Warden procedure was performed for repair of his SSVASD with PAPVR and a left atrial maze with pulmonary vein isolation using cryotherapy was completed for his atrial arrhythmia. Both procedures were completed without complication. A 3D model (Figure 3) was later printed postoperatively to evaluate how surgical repair with patch placement could be improved through practice on a 3D model. Virtual surgery was performed on the model by a cardiothoracic surgeon. This showed how intracardiac patches can be measured, cut, and sewn onto 3D models—all prior to taking a patient to surgery. If employed, the benefits of this protocol would include more precise patch size determination and therefore a cleaner surgical repair as well as better surgical planning with a hope of decreased cardiopulmonary bypass time. Lastly, these models would allow additional training of surgeons who have limited experience in this type of operation.
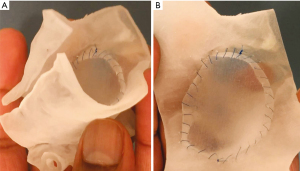
Case 5
Case 5 is a 10-year-old female with dextro-transposition of the great arteries (D-TGA) status-post arterial switch with the LeCompte maneuver. Due to progressive right ventricular outflow tract (RVOT) obstruction, she then underwent subvalvar resection, supravalvular patch augmentation, and branch pulmonary artery patch augmentation with resulting pulmonary regurgitation. A pacemaker was also placed for progressive sinus node dysfunction. She later presented with progressive complaints of fatigue and exercise intolerance with known free pulmonary regurgitation and a non-functioning atrial lead. She was considered for pulmonary valve replacement and an upgrade to dual chamber transvenous pacemaker.
CT scan was performed to assess the RVOT for feasibility of transcatheter pulmonary valve replacement with hopes of avoiding yet another open-heart surgery. Still, the CT did not adequately show if the RVOT and main pulmonary artery anatomy was conducive to Melody valve implantation due to its tortuous track and short main pulmonary artery segment. 3D modeling (Figure 4) was then sought to better understand this area and the feasibility of the transcatheter procedure. The 3D model was taken to the catheterization lab for preprocedural practice of Melody valve placement, which demonstrated anatomy that was conducive to the proposed procedure. Specifically, it demonstrated that valve placement would not impinge into the branch pulmonary arteries and that the main pulmonary artery was of adequate length for stable valve placement without compromising the coronary arteries. The patient was therefore scheduled for cardiac catheterization with transcatheter Melody valve placement—which was successfully performed along with uneventful dual chamber pacemaker implantation. The patient is doing very well, and we were able to avoid surgical placement of the valve.
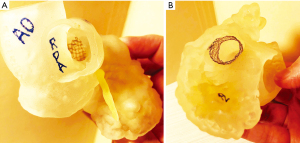
Otolaryngology
The utility of 3D printed models can also be applied to the field of otolaryngology, where key anatomical relationships must be understood with millimeter precision (11). Like CHD cases, patients with congenital airway stenosis frequently require multiple repairs and present many procedural challenges related to the airway’s proximity to major arteries and the small cross-sectional areas involved. 3D printed models have already been implemented in the perioperative planning and surgical simulation of complex airway surgeries such as tracheal resection and reconstruction and sizing endotracheal tubes (11,12). We present 2 cases where3D printed models enhanced the care of patients with congenital airway stenosis.
Case 6
Case 6 is a 4-year-old male with Rubinstein-Taybi syndrome and congenital subglottic stenosis with a history of multiple airway reconstruction surgeries including endoscopic posterior cricoid split and subsequent open anterior and posterior cricoid split. He later presented with copious airway secretions and overnight blood oxygen desaturations requiring supplemental oxygen and was thus evaluated for possible tracheostomy decannulation or revision airway reconstruction. CT angiography of the region showed mild subglottic stenosis in the anterior portion of the trachea; however, it was unclear if the stenosis was causing his symptoms or desaturations. A 3D model of the airway was then printed and used for additional assessment of the stenosis (Figure 5A). The 3D model allowed superior visualization of the airway patency and suggested decannulation of the tracheostomy would provide more benefit than repeat airway repair. The patient subsequently underwent tracheostomy replacement and decannulation without complication. This helped us in understanding the tracheal anatomy and increased our confidence in moving forward with decannulation.
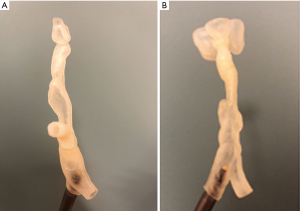
Case 7
Case 7 is a 7-year-old female with tracheal stenosis status-post cricoid tracheal resection then intraventricular laryngotracheal reconstruction with anterior graft. She was later found to have significant tracheal restenosis visualized on CT scan and was considered for tracheoplasty with rib graft or tracheal resection with end-to-end re-anastomosis. For further clarification of the optimal surgical approach, a 3D virtual reconstruction was made, and a 3D model was printed (Figure 5B). The 3D virtual reconstruction illustrated the close anatomic relationship between the site of airway stenosis and the aortic arch and its major branches. The 3D model was used for precise anatomical measurements, revealing the trachea was 2.7 mm at its narrowest portion and 7.8 mm at its widest portion. This information led to the conclusion that tracheal resection with end-to-end re-anastomosis was the optimal approach in this patient—and that the cardiothoracic surgery team was needed to assist with bypass due to the proximity of the neck vessels in the operative field. The patient subsequently underwent tracheal resection with end-to-end re-anastomosis without complication and repeat direct laryngoscopy and bronchoscopy showed drastically improved tracheal stenosis and appropriate healing prior to discharge.
General pediatric surgery
Finally, we highlight the use of 3D printed models in the preoperative planning of general surgery cases, specifically hepatic surgery. Complex liver surgeries such as hepatic tumor resections require extensive knowledge of unique patient anatomy—especially the relationship of the tumor to the surrounding vasculature—in order to achieve optimal procedural outcomes (13). In the last decade, several articles have reported applications of 3D printed models in liver surgery (13-15). We present 1 case of a vascular anomaly and 1 case of solid hepatic tumor resection.
Case 8
Case 8 is a 4-year-old male with Congenital, Lipomatous, Overgrowth, Vascular Malformations, Epidermal Nevi and Spinal/Skeletal Anomalies and/or Scoliosis known as CLOVES syndrome and congenital extrahepatic portosystemic shunt created by an anomalous venous channel between the right internal iliac vein and the junction of the superior mesenteric and splenic veins. If left alone, the shunt would have led to significant sequalae such as liver atrophy and long-standing hyperammonemia in the developing brain. A venogram with transjugular pressure measurements was then performed and upon test occlusion of the shunt, rising portal pressures and vessel size suggested that a controlled surgical ligation was the safest operative approach. Given the anatomical complexity and surgical location, a 3D printed model (Figure 6A,6B) was created to assist with preoperative planning. This led to better understanding and visualization of the shunt and surrounding vasculature which allowed a controlled surgical ligation to be performed safely for the patient without complications.
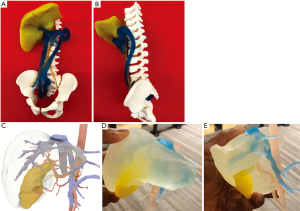
Case 9
Case 9 is a 21-month-old male with a complex medical history who was found to have a liver mass consistent with hepatoblastoma. A 3D model was printed (Figure 6C-6E) and used for preoperative planning. On careful inspection of the 3D model, the vasculature surrounding the mass was delineated and optimal resection dimensions could be planned. An exploratory laparotomy, right hepatic lobectomy, and partial small bowel resection with anastomosis were then performed without complication. A summary table of the benefits of these 3D models in the cases discussed is included in Table 1.
Table 1
Case No. | Age (sex) | Diagnosis | 3D model area in question | Benefit to surgeon | Benefit to trainee |
---|---|---|---|---|---|
1 | 3 months (F) | ISVASD, PAPVR | The relationship of IVC to ISVASD and feasibility of IVC re-implantation into the right atrium | Preop identification of optimal surgical approach and avoiding a baffle | To see how baffle created could cause obstruction |
2 | 17 months (F) | ALCAPA | Feasibility of left coronary artery-aorta baffle through pulmonary artery | Preop planning of a unique surgical approach that would avoid stretching of the coronary artery | Visual evaluation gave more confidence and opportunity to practice virtual surgery |
3 | 18 months (F) | DORV, TGA, LVOT obstruction, subpulmonic stenosis, VSD | Feasibility of left ventricle-aorta baffle through VSD | Preop validation that the proposed surgical approach was achievable when CT data was inconclusive | Visual evaluation gave more confidence and opportunity to practice virtual surgery |
4 | 52 years (M) | SSVASD, PAPVR, atrial fibrillation | Simulation of atrial septal defect patch placement postoperatively | Decreased surgical time | Postoperative simulation of surgical procedure for training purposes |
5 | 10 years (F) | TGA status post arterial switch operation, severe PR | Evaluation of tortuous RVOT and short MPA segment and feasibility of Melody valve deployment. Avoiding coronary artery compression | Investigation of cardiac anatomy and its suitability for transcatheter valve placement | Enhanced spatial orientation |
6 | 4 years (M) | Subglottic stenosis | Clinical significance of mild subglottic stenosis | Determined clinical significance of anatomic abnormality and aided in choice of procedure when CT data was inconclusive | Better understand 3D spatial relationships and opportunity to practice virtual surgery |
7 | 7 years (F) | Tracheal stenosis | Degree of tracheal stenosis and its proximity to major arteries | Preop identification of optimal surgical approach and airway measurements; coordinated involvement of two surgical teams | Opportunity to practice virtual surgery |
8 | 4 years (M) | Congenital extrahepatic portosystemic shunt | The exact location of the shunt and its relation to surrounding structures | Preop planning to understand access point | Better visualization of relationships to surrounding structures |
9 | 21 months (M) | Hepatoblastoma | Assessing vasculature surrounding the mass | Preop evaluation of surrounding vasculature and determination of optimal tumor resection dimensions | Better visualization of the arterial and venous structural relationships |
F, female; M, male; ISVASD, inferior sinus venosus atrial septal defect; PAPVR, partial anomalous pulmonary venous return; IVC, inferior vena cava; ALCAPA, anomalous left coronary artery from the pulmonary artery; DORV, double outlet right ventricle; TGA, transposition of the great arteries; LVOT, left ventricular outflow tract; VSD, ventricular septal defect; CT, computed tomography; SSVASD, superior sinus venosus atrial septal defect; TGA, transposition of the great arteries; PR, pulmonary regurgitation; RVOT, right ventricular outflow tract; MPA, main pulmonary artery.
Discussion
At present, most medical applications of 3D printing come from the surgical subspecialties of orthopedics, maxillofacial surgery, neurosurgery, otolaryngology, cardiothoracic surgery, and general surgery (1,3-5), and include a variety of 3D printed surgical products ranging from anatomical models to surgical guides, prosthetics, and implants (1,4). In addition to improving procedural outcomes, these 3D printed products can serve as valuable learning tools when used for surgical simulation, medical education, and even patient counselling (3,4,7).
Our single-center experience with 3D printing primarily involves the use of anatomical models for preoperative planning and educational purposes. In the preoperative setting, the surgeon’s ability to understand patient-specific anatomical relationships in 3D space is crucial but is often limited by current imaging methods. Here we showcase scenarios where further investigation of these relationships with 3D printed models can be helpful—particularly when complex anatomy is combined with high-risk implications. In each case described, it was clear the models’ tactile and orientation properties had significantly aided the surgeons’ and trainees’ understanding of key anatomical details in 3D space, allowing them to better assess critical areas of concern before planning and executing the optimal surgical approach. When preoperative planning is enhanced in this way, it increases the likelihood for surgical success and may lead to optimal procedural outcomes with a hope of decreasing surgical times, and fewer complications, as demonstrated by the cases in this report.
In addition to improvements in preoperative planning, 3D printed models provide a wealth of educational opportunities. Due to the many pressures on modern surgical training such as work hour restrictions and patient safety regulations, there has been increasing demand for surgical training beyond the operating room (7). This shift in training practices has led to a burgeoning use of surgical simulation modalities which, in addition to 3D printed models, includes human cadavers, live animals, bench-top models, and virtual reality (7). A new age in 3D printing technology sets itself apart by combining realistic human tissue properties with patient imaging data, allowing surgeons to practice a planned procedure, deploy a device, or cut and suture on an individualized patient-specific model before the surgery even takes place. The benefits of this method were highlighted in case 4, where intracardiac patch placement on a 3D printed model allowed surgeons to practice and improve the procedure in a safe and effective manner while simulating patient anatomy with remarkable realism. This type of simulation environment is beneficial to both the experienced and novice surgeon, allowing them to meet their unique training needs while maintaining high-quality procedural and safety standards for their patients (4).
Although medical 3D printing is still in its early development, the surgical applications of this technology promise to improve procedural outcomes, expand learning opportunities, and ultimately enhance the quality of patient care (1,3-5,7). But like most 3D printing literature to date, our case series is limited to subjective, qualitative evidence and more studies incorporating quantifiable outcome measures are needed before definitive conclusions can be made. As we begin incorporating 3D printing into clinical practice, barriers must be addressed such as exorbitant costs and time requirements, limited materials and expertly trained personnel, and the quality and resolution of current medical imaging (1,2,4). Next, there must be government approval via the Food and Drug Administration (FDA) and reimbursement for physicians and ancillary staff to facilitate more widespread adoption of 3D printing in medicine (2,16). Finally, the medical community must weigh the ethical issues surrounding the use of bioprinting and its future in transplant medicine and beyond. As we continue to share our experiences with 3D printing and begin to appreciate its many benefits, we must carefully evaluate and confront these obstacles in order to develop implementation strategies and guidelines that are safe, cost-effective, and clinically useful.
Conclusions
3D printing has emerged as a powerful tool in the surgical setting, supplying surgeons with a multisensory experience of patient-specific anatomy and heightened spatial understanding. Our single-center case series highlights the clinical utility of 3D printed anatomical models in complex cardiac, otolaryngologic, and general surgery cases. When used during preoperative planning, the 3D printed models enhanced our surgeons’ and trainees’ 3D spatial orientation, improved their decision-making when selecting the optimal surgical approach, and benefitted procedural outcomes, possibly decreased surgical times, and fewer complications. By providing an example of surgical simulation, we also showcase how 3D printed models serve as valuable surgical education tools in the modern training environment. While the current report and other qualitative literature sources have generated substantial praise and enthusiasm for this technology, further research involving quantifiable outcome measures and cost-benefit analyses is essential to fully identify and implement the most effective uses of this technology going forward.
Acknowledgments
Funding: None.
Footnote
Reporting Checklist: The authors have completed the AME Case Series reporting checklist. Available at https://dx.doi.org/10.21037/jxym-21-32
Data Sharing Statement: Available at https://dx.doi.org/10.21037/jxym-21-32
Peer Review File: Available at https://dx.doi.org/10.21037/jxym-21-32
Conflicts of Interest: All authors have completed the ICMJE uniform disclosure form (available at https://dx.doi.org/10.21037/jxym-21-32). The authors have no conflicts of interest to declare.
Ethics Statement: The authors are accountable for all aspects of the work in ensuring that questions related to the accuracy or integrity of any part of the work are appropriately investigated and resolved. The study was conducted in accordance with the Declaration of Helsinki (as revised in 2013). The study did not require IRB approval. University of Iowa Institutional review board policy states that case reports or case study are not considered research and are exempt from full application if they are limited to a description of the clinical features and or outcomes and do not contribute to generalizable knowledge. And individual consent for this retrospective analysis was waived.
Open Access Statement: This is an Open Access article distributed in accordance with the Creative Commons Attribution-NonCommercial-NoDerivs 4.0 International License (CC BY-NC-ND 4.0), which permits the non-commercial replication and distribution of the article with the strict proviso that no changes or edits are made and the original work is properly cited (including links to both the formal publication through the relevant DOI and the license). See: https://creativecommons.org/licenses/by-nc-nd/4.0/.
References
- Tack P, Victor J, Gemmel P, Annemans L. 3D-printing techniques in a medical setting: a systematic literature review. Biomed Eng Online 2016;15:115. [Crossref] [PubMed]
- Luo H, Meyer-Szary J, Wang Z, et al. Three-dimensional printing in cardiology: Current applications and future challenges. Cardiol J 2017;24:436-44. [Crossref] [PubMed]
- Langridge B, Momin S, Coumbe B, et al. Systematic Review of the Use of 3-Dimensional Printing in Surgical Teaching and Assessment. J Surg Educ 2018;75:209-21. [Crossref] [PubMed]
- Pugliese L, Marconi S, Negrello E, et al. The clinical use of 3D printing in surgery. Updates Surg 2018;70:381-8. [Crossref] [PubMed]
- Giannopoulos AA, Steigner ML, George E, et al. Cardiothoracic Applications of 3-dimensional Printing. J Thorac Imaging 2016;31:253-72. [Crossref] [PubMed]
- Tejo-Otero A, Buj-Corral I, Fenollosa-Artés F. 3D Printing in Medicine for Preoperative Surgical Planning: A Review. Ann Biomed Eng 2020;48:536-55. [Crossref] [PubMed]
- Badash I, Burtt K, Solorzano CA, et al. Innovations in surgery simulation: a review of past, current and future techniques. Ann Transl Med 2016;4:453. [Crossref] [PubMed]
- Anwar S, Singh GK, Miller J, et al. 3D Printing is a Transformative Technology in Congenital Heart Disease. JACC Basic Transl Sci 2018;3:294-312. [Crossref] [PubMed]
- Moore RA, Riggs KW, Kourtidou S, et al. Three-dimensional printing and virtual surgery for congenital heart procedural planning. Birth Defects Res 2018;110:1082-90. [Crossref] [PubMed]
- Chepelev L, Wake N, Ryan J, et al. Radiological Society of North America (RSNA) 3D printing Special Interest Group (SIG): guidelines for medical 3D printing and appropriateness for clinical scenarios. 3D Print Med 2018;4:11.
- Richard Z, Jackson E, Jung JP, et al. Feasibility and potential of three-dimensional printing in laryngotracheal stenosis. J Laryngol Otol 2019;133:530-4. [Crossref] [PubMed]
- Park S, Ahn J, Yoon SU, et al. Prediction of endotracheal tube size using a printed three-dimensional airway model in pediatric patients with congenital heart disease: a prospective, single-center, single-group study. Korean J Anesthesiol 2021;74:333-41. [Crossref] [PubMed]
- Christensen A, Rybicki FJ. Maintaining safety and efficacy for 3D printing in medicine. 3D Print Med 2017;3:1.
- Witowski JS, Coles-Black J, Zuzak TZ, et al. 3D Printing in Liver Surgery: A Systematic Review. Telemed J E Health 2017;23:943-7. [Crossref] [PubMed]
- Witowski J, Budzyński A, Grochowska A, et al. Decision-making based on 3D printed models in laparoscopic liver resections with intraoperative ultrasound: a prospective observational study. Eur Radiol 2020;30:1306-12. [Crossref] [PubMed]
- Ballard DH, Wake N, Witowski J, et al. Radiological Society of North America (RSNA) 3D Printing Special Interest Group (SIG) clinical situations for which 3D printing is considered an appropriate representation or extension of data contained in a medical imaging examination: abdominal, hepatobiliary, and gastrointestinal conditions. 3D Print Med 2020;6:13.
Cite this article as: Evans AZ, Maldonado JR, Gonzalez N, Wall B, Ashwath R. A single center case series in three-dimensional printing for preoperative planning and surgical education: applications in cardiothoracic surgery, otolaryngology, and general surgery. J Xiangya Med 2021;6:32.